Difference between revisions of "Bioretention: Filter media"
Dean Young (talk | contribs) |
Dean Young (talk | contribs) |
||
Line 29: | Line 29: | ||
!Porosity | !Porosity | ||
|This mixture may be assumed to have a porosity of [[Bioretention media storage|'''0.4''']] unless demonstrated otherwise | |This mixture may be assumed to have a porosity of [[Bioretention media storage|'''0.4''']] unless demonstrated otherwise | ||
− | |This mixture may be assumed to have a porosity of '''0.35''' unless demonstrated otherwise | + | |This mixture may be assumed to have a porosity of [[Bioretention media storage|'''0.35''']] unless demonstrated otherwise |
|} | |} | ||
Revision as of 17:32, 29 May 2020
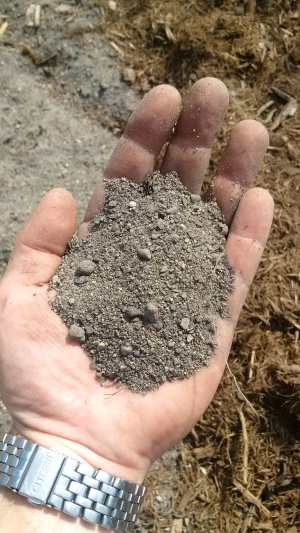
It is recommended that the mixture comprises:
Blend A: Drainage rate priority | Blend B: Water quality treatment priority | |
---|---|---|
Application | Impervious area to pervious area (I:P) ratio greater than 20:1 |
|
Proportions |
3 parts sand |
3 parts sand |
Porosity | This mixture may be assumed to have a porosity of 0.4 unless demonstrated otherwise | This mixture may be assumed to have a porosity of 0.35 unless demonstrated otherwise |
Filter media should be obtained premixed from a vendor and meet all municipal, provincial and federal environmental standards. Mixing of sand, topsoil and compost should be done in a manner that preserves topsoil peds. The mixture should be free of stones, stumps, roots, or other debris larger than 50 mm diameter. Samples of the filter media should be dried, ground and tested to ensure they meet the following specifications:
Characteristic | Criterion | Recommended test method |
---|---|---|
Particle-size distribution | < 25% silt- and clay-sized particles (smaller than 0.05 mm) combined; 3 to 12% clay-sized particles (0.002 mm or smaller) |
ASTM D7928, Standard Test Method for Particle-Size Distribution (Gradation) of Fine-Grained Soils Using the Sedimentation (Hydrometer) Analysis. |
Organic matter (OM) | 3 to 10% by dry weight | ASTM F1647, Standard Test Methods for Organic Matter Content of Athletic Field Rootzone Mixes. |
Phosphorus, plant-available | 12 to 40 ppm | As measured by the 'Olsen' method for alkaline and calcareous soils (common in Ontario). Alternatives include 'Mehlich I or III', or 'Bray', better suited to acidic to slightly alkaline and non-calcareous soils. Results from these are not directly translatable.[1] |
Cationic exchange capacity (CEC) | > 10 meq/100 g | ASTM D7503, Standard Test Methods for Measuring the Exchange Complex and Cation Exchange Capacity of Inorganic Fine-Grained Soils. |
Hydraulic conductivity, saturated (KSAT) | > 75 mm/h; Blend A > 25 mm/h; Blend B < 300 mm/h; Blend A and B |
ASTM D2434, Standard Test Method for Permeability of Granular Soils (Constant Head), when the sample is compacted to 85% of its maximum dry density in accordance with ASTM D698, Standard Test Methods for Laboratory Compaction Characteristics of Soil Using Standard Effort. |
Note that you may choose not to use particle-size distribution as a criterion for acceptance of a filter media blend, but saturated hydraulic conductivity should be one. While information on particle-size distribution and soil texture are useful in selecting plants, the disconnect between hydraulic conductivity and uniformity of gradation makes it far less important than measuring the saturated hydraulic conductivity directly[2]
Sand[edit]
- Coarse sand for LID construction shall be washed clean and free of toxic materials.
- The pH of the sand shall be ≤ 7.0.
- The coarse sand shall have a fineness modulus index between 2.8 and 3.1 according to ASTM C33/C33M, or otherwise meet the gradation below.
Sieve | Percent passing |
---|---|
9.5 mm | 100 |
4.75 mm (No.4) | 95 - 100 |
2.36 mm (No.8) | 80 - 100 |
1.18 mm (No.16) | 50 - 85 |
0.60 mm (No.30) | 25 - 50 |
0.30 mm (No.50) | 5 - 30 |
0.15 mm (No.100) | 0 - 10 |
0.075 mm (No.200) | ≤ 3 |
Topsoil[edit]
- Topsoil may be material that was stripped from the project site and stored in stockpiles for re-use, or material imported to the site from a supplier provided the physical and chemical characteristics are within acceptable ranges.
- Topsoil shall be in compliance with Ontario Regulation 153/04 Record of Site Condition standards for soil quality or as amended through Ontario Management of Excess Soil - A Guide for Best Management Practices.
- Soil laboratory reports shall certify the material to be suitable for re-use on residential, parkland, institutional, industrial, commercial, or community landscapes for the germination of seeds and the support of vegetative growth.
The factors to consider in determining if a topsoil is suitable for use as planting soil for a vegetated stormwater practice, or use in producing a bioretention filter media mixture include the following:
- Must be friable and capable of sustaining vigorous plant growth;
- Must be free from toxic material and roots, stones or debris over 50 mm (2") in diameter;
- Should not have been passed through sieves or screens smaller than 50 mm (2”) to avoid eliminating peds;
- Should have a Loamy Sand, Sandy Loam, Sandy Clay Loam, Loam or Silty Loam soil texture;
- For use as planting soil for a vegetated stormwater practice , the topsoil must contain a minimum of 5% organic matter by dry weight or be amended so, through addition of an organic soil conditioner;
- For use in producing bioretention filter media Blend B (water quality treatment priority), the topsoil must contain at least 9%, and not greater than 36% clay-sized particles and at least 2% organic matter by dry weight.
- Must have a pH of between 6.0 and 8.0;
- Must have a sodium absorption ratio less than 15;
- Must have a cationic exchange capacity greater than 10 milliequivalents per 100 grams (meq/100 g).
Specify that 4 litre samples of topsoil, from each source to be drawn upon, be provided to the consultant for visual inspection, along with topsoil quality test results from an accredited soil testing laboratory, or a quality assurance certificate from the supplier.
We recommend a planting soil or filter media depth of 300 mm to support grasses, 600 mm for shrubs and perennials, and 1000 mm for trees.
Organic component[edit]
This is the first big opportunity to manage phosphorus export from a bioretention or stormwater planter system. While compost is the most common choice, designers working in nutrient-sensitive receiving waters are encouraged to explore the alternatives listed below. Some of these materials may be combined 50:50 with compost to balance providing the nutrients required by the plants with limiting the potential for leaching of excess nutrients.
Compost[edit]
Compost is the most widely used organic component. It's use in bioretention facilities is well established and documented. Low-phosphorus composts should always be sought for use in low impact development facilities, including bioretention. These are typically created from feedstocks including yard, leaf, and wood waste, and excluding manures, biosolids, and food scraps.[3]
Compost Specifications
Even low-phosphorus composts are known to export phosphorus over many years. The use of compost is not recommended in nutrient-sensitive watersheds where phosphorus pollution is a concern. There are a number of alternative sources of soil organic matter which have undergone field studies which have benefits and potential concerns:
Material | Benefits | Concerns | |
---|---|---|---|
Coconut coir[4] | Doesn't leach phosphorus | Must be imported | |
Wood chip | Doesn't leach phosphorus Promotes nitrogen removal from water |
||
Peat Moss | Doesn't leach phosphorus | Must be extracted from natural wetlands | |
Shredded paper (e.g., Pittmoss) | Doesn't leach phosphorus Promotes denitrification |
Wood derivatives[edit]
The 2017 guidance from New Hampshire specifically rules against the inclusion of compost in their bioretention media.[5] Instead they recommend "Shredded wood, wood chips, ground bark, or wood waste; of uniform texture and free of stones, sticks". The use of wood chip has been common in New Hampshire for some time, in this 2006 thesis 20% wood chips (not characterized) were incorporated into all of the test cases to match current practices at the time. [6]
Shredded paper has been tested as an additional source of carbon and as an electron-donor to promote denitrification in a number of successful laboratory and field studies. <ref>
Additives[edit]
Typically these components would make up 5 to 10% by volume of the filter media mixture.
Design innovations to improve water quality treatment performance of filter media mixtures involve the incorporation of additives to enhance retention of reactive or dissolved pollutants. A number of granular amendments have been demonstrated to improve nutrient removal from discharge water in BMPs such as bioretention systems, stormwater planters, absorbent landscapes, sand filters or green roofs.
There are two primary processes involved, chemical precipitation and adsorption. Both mechanisms are ultimately finite, but have been shown in some cases to make significant improvements on the discharged water quality over several years. For instance, a two year STEP research study that compared standard bioretention media, to the same media amended with Sorbtive™ in one plot and iron enriched sand (aka red sand) in another showed statistically significant improvements in effluent phosphorus concentrations from the two media amended plots (STEP, 2019)[7].
Determining when additive enhanced filter media needs replacing or maintenance represents a new challenge for stormwater asset managers, as there are no suitable visual indicators. Erickson et al. (2018) suggest effluent sampling and laboratory testing to identify when enhanced filter media pollutant retention is waning, or periodic sampling and batch (laboratory) testing of filter media to directly measure its capacity to retain the targeted pollutants[8]. Periodic replacement of filter media at inlet locations should be considered as an operation and maintenance best practice to maintain treatment performance.
In our effort to make this guide as functional as possible, we have decided to include proprietary systems and links to manufacturers websites.
Inclusion of such links does not constitute endorsement by the Sustainable Technologies Evaluation Program.
Lists are ordered alphabetically; link updates are welcomed using the form below.
Material | Benefits | Potential concerns |
---|---|---|
Biochar | Renewable Enhances soil aggregation, water holding capacity and organic carbon content |
Currently expensive Energy intensive to produce Some sources say ineffective for phosphorus removal |
Bold & GoldTM | Documented total phosphorus removal of up to 71%[9] | Proprietary |
Iron filings or Zero valent iron (ZVI) | Proven phosphorus retention Retained phosphorus is stable |
May harm plants[10] Removal efficiency declines with increased concentration of incoming phosphorus |
Red sand or Iron-enriched sand | Proven phosphorus removal Also removes TSS |
Poor orthophosphate removal in hypoxic or anoxic conditions |
Smart SpongeTM | Removes phosphorus, as well as TSS, fecal coliform bacteria and heavy metals Non-leaching |
1-3 year lifespan, after which the product is removed as solid waste Proprietary |
Sorbtive MediaTM | High phosphorus removal efficiency | Proprietary |
Water treatment residuals | Waste product reuse | Quality control (capabilities depend on source, treatment methods, storage time) |
- ↑ Sawyer JE, Mallarino AP. Differentiating and Understanding the Mehlich 3, Bray, and Olsen Soil Phosphorus Tests 1. http://www.agronext.iastate.edu/soilfertility/info/mnconf11_22_99.pdf. Accessed August 1, 2017.
- ↑ CRC for Water Sensitive Cities. (2015). Adoption Guidelines for Stormwater Biofiltration Systems: Appendix C - Guidelines for filter media in stormwater biofiltration systems.
- ↑ Hurley S, Shrestha P, Cording A. Nutrient Leaching from Compost: Implications for Bioretention and Other Green Stormwater Infrastructure. J Sustain Water Built Environ. 2017;3(3):4017006. doi:10.1061/JSWBAY.0000821.
- ↑ Rheaume, A., Hinman, C., and Ahearn, D. (2015). “A Synthesis of Bioretention Research in Pacific Northwest.” Herrera, <http://www.modularwetlands.com/new/wp-content/uploads/2015/11/2-Bioretention-Synthesis-2015-DAhearn.pdf>
- ↑ UNHSC Bioretention Soil Specification. (2017). Retrieved from https://www.unh.edu/unhsc/sites/default/files/media/unhsc_bsm_spec_2-28-17_0.pdf
- ↑ Stone, R. M. (2013). Evaluation and Optimization of Bioretention Design for Nitrogen and Phosphorus Removal. University of New Hampshire. Retrieved from https://www.unh.edu/unhsc/sites/unh.edu.unhsc/files/STONE THESIS FINAL.pdf
- ↑ STEP. 2019. Improving nutrient retention in bioretention. Technical Brief. Accessed: https://sustainabletechnologies.ca/app/uploads/2019/06/improving-nutrient-retention-in-bioretention-tech-brief.pdf
- ↑ Erickson, A.J., Taguchi, V.J., Gulliver, J.S. 2018. The Challenge of Maintaining Stormwater Control Measures: A Synthesis of Recent Research and Practitioner Experience. Sustainability. 2018, 10, 3666. https://www.mdpi.com/2071-1050/10/10/3666
- ↑ Hood A, Chopra M, Wanielista M. Assessment of Biosorption Activated Media Under Roadside Swales for the Removal of Phosphorus from Stormwater. Water. 2013;5(1):53-66. doi:10.3390/w5010053.
- ↑ Logsdon SD, Sauer PA. Iron Filings Cement Engineered Soil Mix. Agron J. 2016;108(4):1753. doi:10.2134/agronj2015.0427.